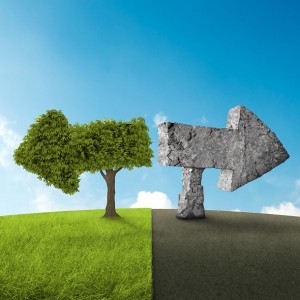
When I hear this question, I think of Lawrence Olivier in Marathon Man probing Dustin Hoffman's teeth to make him reveal the diamonds. Today I repeat the question, but hopefully without the toothache.
Albert Einstein said, “the environment is everything that is not me.” Actually, “me” is definitely part of the environment. It took us a while to understand that, and I will explain the tools we use to get there so you all can explore 3 of the most important questions about us and our environment:
- Are we safe from pollution?
- Is our water supply safe?
- Is our energy supply safe?
The answer to each of these questions is “probably” (did you really expect certainty?), although major changes for water and energy are likely. We'll come back to this, later.
I was an environmental engineering consultant for 45 years. I started my career just after the first Earth Day and I have worked on essentially every type of environmental issue there is. My specific expertise is water quality and I spent the last 30 years of my career working on hazardous waste sites. I used the tools I'm about to tell you about every day.
Environmental Stupidity
Before we get started on the tools, I want to point out some stupid things we do and how forgiving our environment has been.
For starters, consider waste. The United States discharges about 32 Billion gallons of wastewater every day. That's more than one Lake Erie every day. We dump about 250 Million tons per year of garbage. That's Rhode Island 2 ft deep every 10 years. It was worse in the past, but now it's improving, as is our environment.
To date, waste and waste disposal has been necessary, but, some other things we do are not. For example:
- Building cities in the desert.
I worked on a project in Phoenix once where I noticed the City's 500 ft deep groundwater supply wells were designed to draw water beginning from 40 ft below the ground despite there being no groundwater until a depth of 450 ft. This well design seemed unnecessarily expensive, so I asked why they did it that way? The answer was because groundwater was at 40 ft when the wells were installed. Phoenix has “mined” 400 ft of its groundwater. Groundwater “mining” is a term used when more groundwater is used than can be replenished. People in Phoenix have lush lawns and clean their driveways with garden hoses. (I live in water-rich New England and I clean my driveway with a leaf blower.) Is this sustainable?
Las Vegas is spending a Billion dollars on a new water supply intake in Lake Mead because the level of the lake has dropped about 100 feet and the old intakes will be above water.
California cities rely so much on the Colorado River for water supply that this once mighty river now merely dribbles into the ocean.
When it finally does rain in California, building development has denuded the fragile dessert so much that the rain brings devastating mud slides.
200 years ago when we founded our desert cities, our footprint was small enough so that we could muscle the environment to our will. But how long can we sustain life in these deserts now that our footprint has grown?
Learn more about how to become a groundwater protection specialist.
- Nuclear Waste.
Our 60 nuclear power plants in the US generate 2,000 tons of radioactive waste per year. We still have no plan to safely dispose of this waste.
- Ozone Hole.
This is a good story. Stratospheric ozone shields us from deadly cosmic radiation. Without it, life on earth would not be possible. (Ironically, ozone at ground level can kill us.)
In the spring of 1985, we discovered a thinning of the ozone layer over 7 Million square miles of Antarctica. Subsequently, this “hole” grew as large as 11 Million square miles.
The problem was tracked down to our use of Freon-type chemicals. In 1987, 193 nations signed the Montreal Protocol to phase out production of these chemicals.
Ten years later, the ozone layer started recovering. Recovery is slow, however, because these destructive chemicals can linger in the atmosphere for a hundred years. But it seems we dodged a bullet. World cooperation was key and I believe it was only possible because the solution was not disruptive. Conversely, climate change solutions would be disruptive, and the prospect of world cooperation looks pretty dim.
- Climate Change.
Climate change is complex and controversial. Some think it's not true, although 99% of scientists do think it's true. Time will tell; let's consider just a few facts so you can form your own conclusions:
- Carbon dioxide is a greenhouse gas meaning it traps heat on earth, preventing its radiation back out to space. Most excess carbon dioxide in the air is from burning carbon fuels, although making cement contributes 10%.
- Atmospheric carbon dioxide reached 400 ppm last year for the first time ever. (I'll tell you what a “ppm” is in a moment.) In 1960, atmospheric carbon dioxide was about 320 ppm, so today it is 25% higher. Although the earth seems to have 100,000 year cycles whereby carbon dioxide ranges from 200 to 300 ppm, and there are also shorter-term, natural variations, there is no question carbon dioxide today is on the rise beyond earth's historical cycles. No question.
- We know these concentrations from direct measurements in modern times and from measurements in ice cores representing over a million years of history.
(A “ppm” is a unit of concentration that stands for “parts per million,” typically on a volume basis for air but on a mass basis for water. So for air, 1 ppm is like 1 balloon of carbon dioxide mixed among a million balloons of air. Personally, I hate this nomenclature and prefer a more scientific nomenclature of mass per volume, such as “milligrams per cubic meter,” but many people seem to prefer the “parts per…” nomenclature.)
- The earth's temperature is also on the rise - 1 degree centigrade over the last 100 years to about 58 degrees on average. Doesn't seem like much, you say? This small change has caused Greenland's ice shelf and glaciers throughout the world to recede (melt) in some cases by more than a mile. That's a fact.
- Sea level is rising about 0.1 inch/year. That's another fact. Small you say? The Economist magazine reported (Jan 17, 2015) that experts predict $100 Billion of US real estate will be underwater by the end of the century. A warming earth causes the oceans to expand and melting ice creates more water.
- “Heat of fusion” is a scientific principle that is important for the earth's ice. It takes 1 calorie to heat 1 gram of water 1 degree, but the phase transition from ice to water takes an additional 80 calories without any temperature change. That's called water's heat of fusion. This means that once ice is melted, it will take a lot of cold (absence of heat) to get it back. Making new ice caps will be like putting the genie back in the bottle. In addition, once the ice melts, additional heat will make temperatures zoom.
- The oceans absorb carbon dioxide from air. Optimists hope this will take care of the excess. No one knows yet.
These are indisputable facts - atmospheric carbon dioxide is the highest ever, temperature is rising, and the ocean is swelling. Skeptics' primary argument about these facts is that they are simply natural variations. Almost all scientists disagree and argue these changes are beyond normal. But forget that part of the debate. Whether they are natural or man-made is not important at this point. The important point is how will they affect our environment? No one knows. Some models predict that ocean currents, like the Gulf Stream which pumps heat from the equator north, will stop and cause an ice age. Other models predict extreme weather - wet will get wetter and dry will get drier - and changes in agriculture. But today all the causes and effects of climate change remain fuzzy so the political world is not willing or not able to come to grips with precautionary action. So instead of “prevention,” most strategies today focus on “adaptation.” But how much adaptation will be required? What will life be like? No one knows.
- Landfill black holes
Although this might fit more into the category of “scary” than “stupidity” I want to tell you a story about just 1 landfill that closed around 1990. I worked on this landfill in California. Because it wasn't built with the right safeguards in the first place (there were no such regulations when it opened), the operation and maintenance budget for this landfill is currently about $25 Million/yr. Just one landfill. Some of that budget is for studies of how to improve things, but it is unclear to me that the landfill really poses a risk despite the fact that there are millions of gallons of waste in it. The real point of concern is the $25 Million/yr operating cost for a closed landfill. Multiply that times all the other landfills that might need such annual spending and you realize how little money we have left over for other environmental needs.
Environmental Basics
Despite such gloom and doom, I have repeatedly been amazed at the environment's resiliency. And the better we measure it, the better we understand and can manage our part in it.
So how do we consider the environment? The environment might be described broadly as:
- Air, Water, and Soil
- Outdoor and Indoor
Outdoor and indoor air, water, and soil are called “media.” Indoor “soil” is more commonly called “dust” unless you live in a pig pen, of course. Acting on these media is all kinds of chemistry, physics, and biology.
We are the only part of the biology that can destroy it all, but there are many other forms of biology playing important roles in our environment including bacteria, algae, ants and trees, among others. All this biology is affected by 4 important factors of chemistry and physics:
- Oxygen
- Enzymes
- Energy
- Toxicity
Oxygen
Oxygen plays a central role in the environment. Aerobic organisms, like us, fish, and many bacteria, need it to live, while anaerobic organisms die from it. Most of the healthy world is aerobic but two important anaerobic microbial reactions that occur under your nose are fermentation and digestion. Healthy water has about 9 mg/l of oxygen dissolved in it and fish love that. Our understanding of dissolved oxygen in water awakened in 1888 when Ludwig Winkler invented a convenient way to measure it. 1925 saw another great leap when 2 US Public Health scientists, Streeter and Phelps, developed a model describing the balance of oxygen in streams caused by decaying pollution and atmospheric reaeration.
From those two eurekas came our entire science of sewage treatment and surface water management. There are 16,000 sewage treatment plants in the US, all treating and discharging sewage at levels prescribed by this science and regulated by US EPA so that acceptable water quality is maintained in most of them. As described later, wastewater discharge limits are based on what we call “waste load allocation” calculations using versions of the Streeter and Phelps model while microorganisms mop up the rest in a process we call, “natural purification,” also based on the principles described by Streeter and Phelps.
When too much waste is discharged to water, oxygen levels deplete from its decay, fish can die, and nasty anaerobic microorganisms take over. The water becomes stinky - that's a technical term - because those anaerobes spew out sulfur and ammonia.
In the early 1900s up to the 1970s, our rivers and lakes were headed for real trouble. Population increases caused excessive waste discharges which put too much pressure on natural purification. There was little wastewater treatment and much to learn about pollution. Water-borne diseases from sewage, such as typhus and dysentery, were rampant and rivers even caught on fire. Lake Erie was dying, meaning detergent-laden phosphorus-engorged algae blooms decayed and depleted the lake-water's oxygen. That process is called eutrophication. After decades of debate and improved scientific understanding, the US finally established a federal law, the 1972 Clean Water Act, which turned the tide.
Water-borne typhus is now a thing of the past and more and more miles of rivers and acres of lakes are becoming swimmable and fishable again. Our waters are becoming healthy with oxygen.
Enzymes
Enzymes are chemicals in plants and animals that facilitate chemical reactions. It is amazing how so many things would not happen without enzymes. Most of these enzymes exist in microorganisms, which can be viewed as little chemical reaction factories. Sewage treatment and receiving water natural purification are two examples of critical microbially-mediated enzyme reactions. For example, one of the most common processes for sewage treatment is called the “activated sludge” process. In this process we let bacteria and fungi grow rampantly in tanks we feed with sewage and air. This “activated sludge” of microorganisms then breaks down the waste molecules with enzyme reactions much more efficiently than we could otherwise do with man-made chemical reactions.
Enzymes also play a role with algae's creation of oxygen and forming the base of the food chain. Enzymes also play a role in microorganisms taking nitrogen from the atmosphere and converting it to forms of fertilizer. Our environment would not be hospitable to humans without enzymes.
Energy
Energy plays a fascinating role in the environment because it both hurts and helps. Radiation energy can kill, but energy can be used to desalinate life-giving water. Engineers use a tool called mass and energy balances to describe almost everything. These are equations based on the notion that mass and energy are never created, they just change forms.
Mass and energy balance analysis has been useful to learn how the environment works. Entire ecosystems are thus described by tracking the energy flow from sunlight in primary production (e.g., algae growth) to primary consumers (e.g., bugs that eat algae) to higher consumers all the way to the top, such as sharks in the ocean, and back through scavengers and degraders. In Watergate we followed the money, in water we follow the energy.
Toxicity
Everything is toxic at some level. To quote Paracelsus, a 16th century rogue, “the dose makes the poison.” Toxicity is important to the environment for 2 reasons - human health and environmental process functioning. For example toxic chemicals in a discharged wastewater might prevent the bugs in a stream from doing their thing with natural purification. For the former, human health, I will describe our first tool of environmental analysis - risk assessment.
Environmental Tools
Risk assessment was first formalized in the 1980s when the federal Superfund law started cleaning up hazardous waste sites. Recognizing that zero contamination was neither practical nor necessary, we needed to predict just how much contamination was tolerable, and risk assessment became the tool to do that - with it we could ask how clean is clean enough? That question was the crux of my career. Is it safe?
Today it is routine for environmental scientists to consider the safety from contamination using human health risk assessment and ecological risk assessment. The latter name is actually a misnomer because ecological risk assessment examines only selected organisms, not ecosystems, using human health risk assessment methods.
Although there are more complicated definitions, the simplest way to think of human health risk assessment is in 3 parts - 1) to the body; 2) into the body; and 3) inside the body. Prediction of how contaminants get to the body is a science we call “fate & transport.” Prediction of how much gets into the body is a science we call “exposure assessment.” Predicting what happens when a contaminant is in the body is a science we call toxicology. Exposure assessment is a combination of posing “exposure scenarios” - how many times a week someone takes a shower and for how long, for example - as well as assigning exposure factors, such as how much chemical gets absorbed through skin, for example.
Fortunately, we don't have to make this stuff up every time we analyze it. There are regulatory guidance manuals on exposure factors, as well as on the whole risk assessment methodology. EPA maintains a database of toxicity factors, now on the internet and called IRIS (Integrated Risk Information System). More on IRIS, later. The science of “fate & transport” is perhaps the most complex part, but it is based on well-defined engineering principals, such chemical solubility and fluid transport mechanisms, and on mathematical models that facilitate the calculation of complex processes of cause and effect. With this science we predict concentrations at locations of interest distant from a contaminant discharge point, i.e., a “source.” I will spare you further details, if you will spare me from snoring. Just rest assured that risk assessment calculations are reproducible, defendable from scientific research, and are usually reviewed for reasonableness and accuracy by peers or regulators.
Perhaps the best way to explain risk assessment further is to demonstrate it with a simple example. Consider a waste discharged to a river used for drinking water supply.
First the fate and transport part:
- I must determine what chemical contaminants are in the waste.
- I must then determine the concentrations of those chemicals at the point of water supply. To do this I need to know how much chemical is discharged and how it dilutes. Then I must consider possible transformations, such as chemical reactions or microbial effects. Then I must consider phase changes, such as attachment to particles that are removed by sedimentation or volatilization to the atmosphere. After considering all of these processes, I can predict the concentration of each pertinent chemical at the drinking water supply point.
Now for the exposure part:
- The exposure analysis predicts the dose. “Dose” is the amount of contaminant that gets inside the body.
- First I pose all the possible exposure scenarios. In this simple example, 2 exposure scenarios will be considered: drinking water and showering. I will ignore others, like getting water on your hands when you wash your car. These others are probably minor and you've got to draw the line somewhere!
- For each exposure scenario, I must assign exposure factors. For example, a standard assumption for the drinking water consumption rate is 2 liters per day. For the shower scenario, I must define how long the shower lasts, how many times a week we shower, etc. Going back to fate & transport analysis, I must also predict how much contaminant volatilizes during the shower. For the shower scenario, I will consider inhalation but ignore dermal absorption.
- The dose result is the mass of contaminant entering the body, grams per day per kilogram of body weight, for example, through each pertinent “exposure route,” such as ingestion, inhalation, or dermal absorption.
And, finally, the toxicity analysis:
- Risk is calculated by multiplying the dose times a toxicity factor. Two kinds of health effects are evaluated, cancer and noncancer (e.g., kidney disease) and different toxicity factors exist for each “endpoint.” How risk is expressed will be described in a moment.
- Toxicity factors are developed in the laboratory by exposing lab animals to various doses and noting their health effects. Using scientific knowledge about toxicity and after applying safety factors to account for the fact that most people are not rats, these results are translated into human toxicity factors.
- EPA takes what their scientists believe are the best developed toxicity factors and maintains them in a constantly updated internet database called IRIS, as mentioned earlier.
- It is possible to challenge IRIS values and some contaminants are not in IRIS, both of which would require a toxicologist's expertise, but in most cases this part of risk assessment is merely looking up appropriate values in IRIS. More or less monkey work.
- Cancer risk is expressed differently than noncancer risk. Cancer risk is expressed as an incremental probability of getting cancer from the exposure - “incremental” meaning above and beyond other, “daily” cancer risks. Non-cancer risk is expressed as a ratio against a level considered safe. This is called a “Hazard Quotient.” When evaluating more than one contaminant, these Hazard Quotients are added together, as long as they apply to the same health effect, into a “Hazard Index.” A Hazard Quotient or Index less than 1 is considered safe because it is less than what is considered a safe level. A cancer probability is a little trickier because it is subjective. Our everyday risk of cancer is about 1 in 3. So what additional cancer risk due to the contaminant exposure is tolerable? For Superfund sites, EPA says 1 in 10,000 and after a cleanup, incremental risk must be no larger than 1 in 1,000,000. Comparing this regulatory threshold to the 1 in 3 we all live with from all other causes, one can see that we are tolerating very little risk from hazardous waste sites.
- As an aside, you should know that EPA's advisory safe level for radon in your home is slightly higher than its threshold for action at Superfund sites. Said another way, EPA may require a multimillion dollar cleanup at a Superfund but advises you that the same risk is ok in your home.
The regulatory world generally considers safety to be within a risk range of 1 in 1 Million to 1 in 10,000. To put this in perspective, consider the risks of some daily events:
- The risk of being struck by lightning is 1 in 12,000
- The risk of dying in a car accident is about 1 in 7,000
- The risk of dying in an airplane crash is about 1 in 11 Million
Although it should be clear that risk assessment is a valuable tool, it is important to understand that it usually overestimates risk under current practice because:
- IRIS toxicity factors have built in safety factors, sometimes up to a factor of 1,000; and
- Exposure scenarios usually are overly extreme.
- For example, I once worked on a Superfund site where the regulators required us to consider a 1 year old infant's exposure to PCBs in sediments 4 ft deep. Resisting, I argued that the infant's health problem in that scenario would not be PCBs.
- EPA rules require postulating what is called a “Reasonable Maximum Exposure” (RME). Generally, there is little that is reasonable about what is required to consider.
What all this means to you if you find yourself in a potential exposure situation is that:
- The calculated risk number is probably much higher than what you will experience; and
- You need to consider if the exposure scenarios posed in the risk assessment apply to you.
One last point I'll make about risk assessment is one that bugged me for the 25 years that I ran a risk assessment consulting firm. In Superfund and other regulatory programs, we make decisions based on estimated risk. It should be risk vs benefit. Risk-benefit analysis is a basic tool that I learned in engineering school. We all make risk-benefit decisions daily. For example, we usually decide the benefits of driving our cars outweigh the risks from a car crash. Although a one-sided risk only analysis might be appropriate for examining existing conditions (we call this a “baseline risk assessment”), a risk-benefit analysis is most appropriate for deciding what needs to be done. This is currently not done properly in Superfund and I believe much money is wasted as a result.
Now you know how to think about contamination. The next tool is about how we measure it.
Measurement
There are 2 steps to measuring environmental contamination - sampling and analysis.
- The key to good sampling is to ensure it is representative. Generally we take a few bottles of stuff, soil or water for example, and expect it to be representative of a large area or long period of time. There are textbooks written about how to do this properly, both in terms of the appropriate number of samples and of their location. Random, grid, and targeted sampling are 3 of the textbook strategies.
- One thing to keep in mind for sampling is whether the same contaminant of concern is present due to other causes. We call this “background.” Ignoring it can be misleading and expensive. Years ago at Love Canal, frenzy was triggered because indoor air benzene results ignored the background caused by gasoline from a motorcycle repair in the basement.
- After collection in the field, samples are sent to a laboratory for analysis. Labs have sophisticated instruments for this called gas chromatographs, mass spectrometers, atomic absorption, and inductively coupled argon plasma, to name a few. Samples are prepared and analyzed with these instruments under strict quality control steps prescribed by very detailed methods published by regulatory agencies and trade groups.
- Now we have the data, but don't take the results at face value. There are many reasons environmental data might not being accurate or precise. I spent much of my career dealing with this issue and offer 2 rules of thumb:
- Results are never exact, but for good data they are close;
- High values are close enough but values close to the detection limit may be very wrong in a relative sense.
When I'm out of thumbs, I usually assume environmental data are good within a factor of 2.
Standards and Criteria
Measurement tools allow us to define contamination and risk assessment is the tool to interpret what it means. A more generally applicable interpretation tool is to compare concentrations to criteria and standards. Standards are enforceable regulatory numbers. Criteria are suggestions based on the pertinent science. Both are often derived using risk assessment.
The United States has standards and criteria for many environmental media we encounter every day. For example:
- Drinking water safety. EPA maintains a list of MCLs, “Maximum Contaminant Levels,” for dozens, perhaps hundreds of compounds or parameters (e.g., radioactivity or bacteria) in publicly supplied drinking water. In addition, there are testing and reporting rules for every such water supply. Water districts over a certain size must make those testing results available to you annually.
- As an aside, let me tell you the results of a water quality survey EPA once did on bottled water. They found one-third of the waters tested failed drinking water standards.
- Air quality. EPA maintains air quality standards (NAAQS, National Ambient Air Quality Standards - the environmental field is full of acronyms, but nowhere near the Navy's lexicon) for 6 “criteria pollutants” - lead, particles, sulfur dioxides, nitrogen dioxides, carbon monoxide, and ozone in ambient air along with rules for measuring them throughout the US. These are the most important general air quality parameters influencing smog, acid rain, and general health effects. In addition, EPA regulates emissions from potential sources of 189 Hazardous Air Pollutants (HAPs) that might have specific health effects.
- Water Quality (lakes and streams). EPA maintains a list of ambient water quality criteria and states offer quality standards for targeted stream classifications. Also, all wastewater discharges are regulated so as to ensure that stream standards are met. This is done through a program called NPDES (the National Pollutant Discharge Elimination System), which requires a permit for every pipe in the US discharging to a receiving water. Contaminant limits are customized to each source of each pipe after considering the needs for receiving water quality. Receiving water quality is defined for each water body in the US, including groundwater, by agencies assigning a classification to each. Classifications, which are usually designated alphabetically (“A, B, C, etc.”), define the water quality goal for that water, such as drinkable, fishable, contact recreation, and in some cases even limited to waste conveyance. EPA's permit program for what is called point source pollution, i.e., from industries and city sewage treatment plants is quite mature and sophisticated. Its permit program to control non-point sources, such as stormwater runoff, is technically more difficult but is improving every year.
So to understand if we are safe from pollution, environmental scientists measure the pollution, consider its fate & transport, consider its potential health impacts, and compare it to standards and criteria, all while considering the myriad of factors that interfere with interpretation. Now let's get back to that question, is it safe?
Question 1. Are We Safe From Pollution?
Most likely, yes.
The water we drink, the air we breathe, and our rivers and lakes all have their quality regulated in one way or another. One US debate in the early 20th Century was whether to allow unfettered waste discharge offset by strict drinking water treatment regulation as opposed to regulation of waste discharges so as to protect drinking water supplies. We pretty much went the first route because of the invention of chlorination until the 1970s, when we realized we needed both - discharge regulation along with strict water supply regulation and treatment just to keep up.
Environmental quality regulations are based on the most recent, credible scientific analysis. Sometimes new scientific understanding demands an update to a standard. For example, in 2008, the air quality standard for lead decreased from 1.5 to 0.15 ug/m3 after a debate lasting over a decade. These debates try to resolve the scientific research with safety, costs, practicalities, and an appropriate margin of safety. Rarely is the path clear.
So I believe our general environment is safe, but there are some specific potential threats still worth considering further. For example:
- Radon in homes. Even low levels typically found in homes can cause lung cancer.
- Electromagnetic Fields (EMF), such as from high voltage lines. The jury's still out, but this is unlikely to be a serious risk in most cases. Interestingly, having your bed against a wall with a refrigerator on the other side might offer the largest risk.
- Chemicals in products. In my opinion, this is the most important risk of contamination in most people's lives. Look at the labels on your cleaning products and you will see up to 99% “inert ingredients.” That means 99% is inert for the purpose of the product but not necessarily for health effects. Chemicals in both cleaning and personal care products volatilize into your home's air and absorb through your skin, but no one regulates or even evaluates these potential risks.
In my opinion, chemical product safety is the last frontier for “the environment” and it's a big one.
Question 2. Is Our Water Supply Safe?
Water For People, a charitable organization, claims that several Billion people in the world spend 4 to 8 hours a day just getting water. Fortuitously, the US doesn't have this problem, even though we use 350 Billion gallons per day of fresh water, 21% from groundwater and the balance from surface water. Some other interesting statistics for our US water supply (2010) are:
- Domestic use is 28 Billion gallons per day, 87% from public supplies and the balance self-supplied.
- 14% of domestic supply is lost to leaks. Of the balance is for toilets use 27%, washing uses 22%, showers use 17%, and faucets use16%.
- Our average daily percapita water useage is 88 gallons. When I started my career, the standard assumption (such as for sewage treatment plant design) was almost twice this.
- California uses the most water, almost 40 Billion gallons per day, with about 60% of that for irrigation.
- Texas is second (25 Bgd), Florida third (15 Bgd) and Rhode Island last (0.2 Bgd)
- Water withdrawals (everything, fresh and saline) grew from 180 Bgd in 1950 to 420 BGD, teetered around that until 2005 and has declined to 355 Bgd in 2010. During this time, US population doubled from 150 Million to 300 Million so various efficiencies have been effective.
- Water Reuse (from wastewater back to beneficial use) is about 3 Bgd. California and Florida are the largest reusers; add Texas and Arizona and 95% of US reuse is accounted for. NRC estimates that up to 30 Bgd could be reused.
- The US has about 10% of the world's current desalination capacity, which is 21 Bgd.
- Desalination is not the “get out of jail” card you might believe because it costs energy. It takes about 1 kWhr to desalinate 100 gallons of seawater. MIT is working on a new desalination membrane that produces energy as a byproduct.
In general, the water picture for the rest of the world is different than for the US. Some of this is out of local necessity. For example:
- Israel reuses 70% of its domestic wastewater, Singapore 30%, Saudi Arabia 16%, and Australia 8%.
- Saudi Arabia has the largest desalination capacity at 3 Bgd, with the Middle East overall at about 6.5 Bgd, or 30% of the world total.
- Globally, water consumption has tripled over the past 50 years. This is partly due to population growth but also to development.
- The lack of supply infrastructure and poor water quality threatens many developing countries.
So what about this second question of whether there will be enough water? A precise answer is not possible because it depends on how development occurs in the future and on energy, which I will discuss next. Future water wars are possible, but not inevitable. Let's explore the question by considering a range of developing nations - China, Nigeria, and Saudi Arabia.
China
China is roaring with modern development and its water supply issues are severe, as a result. For example:
- China needs 146 trillion gallons of water per year - 12% domestic, 65% agriculture, and 23% industry.
- China is mining its groundwater in 160 areas, totaling 2.6 trillion gallons per year mined. 50 cities suffer from land subsidence as a result.
- 80% of China's water resources are in the south of China, which is not aligned with its needs. A north-south water transfer project is underway. Pure muscle.
- China depends on some water originating and thus possibly “owned” by other countries including Tibet, Vietnam, Cambodia, India, and Russia.
- Extensive infrastructure projects including supply, water quality, desalination, and water reuse are underway in China. Still, 300 Million people in China have no access to clean water. 60% of China's surface water is so polluted it cannot be used for water supply.
- About 400 of China's 660 or so cities face water shortages, including 30 of its 32 largest cities.
- Beijing needs 2.6 Bgd but has safe supplies of only 1.5 Bgd.
- Beijing uses twice the amount of water percapita as does Melbourne, Australia. Clearly water conservation will be part of China's future.
Bottom line, China has great infrastructure capability, but severe water supply issues due to population and development growth. Depending on internal fixes, China's future may include conflicts with its neighbors about water.
Nigeria
Nigeria should be a benchmark for the developing African continent, due to its oil wealth, but it has an abdominal water supply. For example:
- Access to any kind of improved water is only 50% in rural areas and 75% in urban areas.
- 80% of government water supplies in small towns are non-operational.
- Lagos, Nigeria's largest city with 12 Million people in the area, has treated water supplies totaling only about 150 Mgd. (At the US average of 88 gpdpc, this would mean 14% of the population is served.)
- The water is there. Nigeria's daily water replenishment to surface and groundwater is 220 Bgd. With proper management much of this could become sustainable water supply. Nigeria's population is 174 Million, so this supply potential could easily support an equivalent to today's US average. But it's not happening.
Bottom line, Nigeria's problem is infrastructure. Even if it wanted to fight about or steal water from its neighbors it couldn't supply it to its people. Many developing nations are in the same boat.
Saudi Arabia
Saudi Arabia has only 24 Million people, but it represents a rich desert nation that can easily buy its water and distribute it to its people. Saudi Arabia has no permanent rivers or lakes and hardly any rainfall. Much of its water supply comes from groundwater and desalination. For example:
- About 50% of drinking water comes from desalination, 40% from groundwater mining, and 10% from intermittent surface water in the mountainous southwest.
- Some groundwater aquifers have dropped over 450 ft over the past 25 years.
- The Saudi government operates 27 desalination plants generating 3 BGD.
- Municipal water use is 1.5 BGD, 9% of total (another 88% goes to agriculture).
- Water reuse and conservation have decreased new water demand by up to about 40%.
- Water rates in Saudi Arabia are about $0.40/1000 gal. Compare this to Boston's rate of $6.50/1000 gal and to $4/1000 gal for desalination. Clearly the Saudi government is subsidizing the cost of water.
- From 1975 to 2000, Saudi Arabia invested $100 Billion in water supply and sanitation infrastructure, 1.5% of GDP, among the highest relative spending in the world. In 2010, it was spending $2 Billion/yr.
Bottom line, Saudi Arabia, one of the scarcest water zones in the world, supplies water almost for free. It can do this, relying heavily on desalination, because it is energy rich. But can this country and other governments that subsidize water do it forever?
These current examples span a range of conditions and suggest a future that will vary depending on local conditions, including possible conflict, poor distribution limiting growth, possible community collapse after subsidy collapse, and buying out of problems with excessive energy.
This brings us to our third question.
Question 3. Is Our Energy Supply Safe?
Saudi Arabia is a perfect example of the inextricable connection between water and energy. For many countries, a future with energy problems will mean a future with coincident water problems. Whether life can be sustained in such countries remains to be seen, but globally, water-rich countries may be left only with energy problems. And there will be energy problems.
First a lesson on units of energy. Today we talk in Quads. A “Quad” is a quadrillion BTUs. A BTU (British Thermal Unit) is a basic unit of heat, which is a form of energy. The US uses 95 Quads of energy per year. In 1980, it used 75 Quads. Another basic unit of energy is electricity in terms of “Kilowatt-Hours (kWhr).” Converting all of its energy forms into this unit, the US uses 28 Trillion KWhr per year. My house uses 4 billionths of a percent of this.
The world uses about 530 Quads per year. There are about 9,000 Quads of proven oil reserves, 6,600 Quads of natural gas, and about 18,000 Quads of coal reserves. At the current consumption and proven reserves, this gives about 63 years of carbon energy. Let's more than double that to 150 years to account for unproven reserves. That's our carbon energy future, 150 years, ignoring growth and climate change.
After our carbon energy is used, there are 2 likely replacements - nuclear and renewables. For the latter, let's eliminate bio. If all the annual production of biomass in the world were converted to energy it would satisfy about 20% of the world's energy needs and leave us with no food. That leaves primarily solar, wind, and water power.
- In 2011, 9% of US energy was supplied by renewables - 1% by wind, 0.2% by solar, 3% by hydroelectric, and 2% by wood, among others.
If hydroelectric is about as developed as it's going to get, that leaves solar and wind for our renewable energy future. Let's consider solar (photovoltaics) first.
- About 2 Million Quads of solar radiation hit the earth each year. That's 4,000 times more than we need for the world. 1,200 times, based on sun hitting just land.
- Solar panels operate at about 15% efficiency. Let's hope for 30%.
- At this higher efficiency yet to be achieved, 600,000 Quads or 1,000 times our needs are available if we covered the entire earth with solar panels, 300 times on just land. Said another way, to satisfy all the world's energy, we would need to cover 200,000 square miles with solar panels.
- A typical solar panel is 18 square feet so that would take 300 Billion solar panels.
- I put 20 solar panels on my house for $35 K. So we are talking about a capital investment of $10 Quadrillion for solar to satisfy the world's energy demand. That's 600 times the US GDP.
- If economies of scale saved us a factor of 10, that leaves a total cost of 60 US GDPs.
- Serious stuff. And we haven't even considered the practicality issues. Read more about solar power pros and cons.
Wind energy has its own unique requirements:
- Cape Wind, in my backyard, will cover 24 sq mi of nearshore ocean with a rating of 450 Megawatts. (For comparison, this is about half the size of a nuclear power plant).
- At this potential for 6.7 Billion KWhr, 110,000 square miles would satisfy the annual US energy demand. This is about the size of Colorado. Read wind power 101.
Despite these scary statistics, there is no question in my mind that solar and wind have a permanent place in our energy future. Nuclear power is probably the other piece. Nobody wants a nuclear power plant in their backyard, but new technologies are making them safer to consider. Current plants are nuclear fission based on splitting uranium; fusion - hydrogen to helium - which generates 4 times more power, is our hope for the future.
- There are about 60 nuclear power plants operating in the US today. (They generate 2,000 tons of radioactive waste/yr and we have no plan dealing with this.)
- About 8% of US energy, 8 Quads, is currently supplied by nuclear power, but a new nuclear plant has not been built in the US for about 20 years.
- A new, 1,000 MW nuclear power plant might cost $6 Billion to build. Thus it would cost about $12 Trillion to convert the world to nuclear energy. Cheaper than for solar, but maybe not after we pay to manage the waste properly.
These examples demonstrate that there will be incredible costs and practical concerns about energy after carbon's demise. But what is our choice?
So let me finish by summarizing my thoughts on the 3 safety questions:
- Are we safe from pollution?
- Generally, yes. The quality of the air we breathe and the water we drink continues to improve and is fairly well regulated. In most cases, there is little actual risk from hazardous waste sites. Perhaps the largest potential risks we face are: 1) radon in our homes; 2) household chemicals; 3) radiation; and 4) climate change.
- Are we safe because the earth will have enough water?
- The uneven distribution of water will cause problems, such as potential aggression by powerful countries like China and economic upheavals within areas of some countries like the southwestern US. Safe water distribution will limit development in much of the world. Water will become increasingly critical for agriculture to meet growing population needs. But overall, improving water reuse and the potential for desalination if we can solve the energy equation offer long term hope for water safety.
- Are we safe because the earth will have enough energy?
- This is perhaps our most difficult issue. Fracking has given the US a reprieve, but don't be fooled no matter what that blond lady on television commercials tells you. There are absolute limitations to our carbon energy future. Some combination of renewables and nuclear power is our most likely solution, but there will be great costs and there are risks. Some kind of a “technology fix” would be nice, perhaps from dark energy. The safety issue about energy if we don't crack the code may not be extinction, but it will be the end of life as we know it.
So drink safely and build a windmill.
Other Lectures by Dr. Neil S. Shifrin
- Environmental Perspectives
- Environmental Management and Its History
- Is It Safe? Raising Environmental Questions (Currently here)
- Environmental Perspectives - July 30, 2018
- Environmental Management and Its History - July 31, 2015
- Is It Safe? Raising Environmental Questions - April 10, 2015